
Research Projects
[1] Synthesis and development of cheap but efficient photocatalysts for hydrogen generation through water splitting and pollution remediation
An electrospinning technique will be applied to fabricate core-shell and hollow nanofibers catalysts and anion and cation doping will be utilized to engineer the bandgap to absorb visible light and catalyze H2 formation.
[2] Synthesis, characterization, and property evaluation of inorganic heterogeneous catalysts for biodiesel production
A bi-functional catalyst consisting of decorated core-shell type nanofibers and their aerogels will be studied for biomass conversion to produce biodiesel.
[3] Synthesis and characterization of efficient cathode materials for Li-air batteries
An ultrasonic spray pyrolysis technique will be employed to prepare efficient bi-functional perovskite/carbon composite materials as Li-Air battery cathodes.
Photocatalysis:
The current drive towards developing efficient photocatalysts demands the engineering of materials. A wide range of inorganic and organic materials have been explored as catalysts to harness solar energy in applications such as hydrogen generation, chemical conversion, and chemical degradation. The choice of material depends on many variables including band gap energy (BG), stability under irradiation, cost effectiveness, and easy preparation. Additionally, lowering the recombination probability of photogenerated electron-hole carriers and making them readily available for water oxidation and reduction reactions are of high importance.
The photocatalytic watersplitting using semiconductor nanomaterials is initiated by the direct absorption of a photon, which creates electrons and holes (photo carriers). Then, the photo carriers move to the surface of the semiconductor (photocatalyst) and react with water provided the potential difference of this reaction exceeds 1.23 eV. The electrons excited from valence band (VB) to conduction band (CB) reduce H+ ions into H2 gas (HER) while holes oxidize water into O2 (OER). This process is governed by intrinsic factors of photocatalyst such as the position of energy bands, band gap, crystallinity of material, availability of co-catalysts etc.
Among these factors, band gap plays a vital role and determines whether photocatalyst absorbs visible light. Therefore, manipulation of band gap energy of the particular photocatalyst by engineering material properties or by combining with another photocatalyst that absorbs in the visible region is required. Despite the fact that a wide range of photocatalysts have been studied, most of them absorb in the UV region, which accounts for ~5% of the total solar spectrum. If visible solar energy is to be efficiently harnessed, a photocatalyst with lower band gap (<3 eV) must be developed in order to facilitate absorption in the visible-range, which accounts for approximately 40% of solar spectrum. It is quite challenging to develop low band gap semiconductors with sufficient stability and surface properties that lead to higher activity. Additionally, it is also challenging to keep photo carriers from recombination, which is equally responsible for low catalytic activity of photocatalysts. Therefore, in our research, we plan to address strategies to manipulate photocatalyst structure, to develop efficient co-catalysts, and to alter catalyst surface in terms of surface area. Photocatalyst structure modification will be achieved by synthesizing one-dimensional (1D) heterojunction nanofiber photocatalysts. Surface modification of heterojunction nanofiber photocatalysts will be conducted by assembling 1D heterojunction nanofibers into three-dimensional (3D) architectures (aerogels) and by developing cost-effective core-shell type monolayer and alloy co-catalysts.


SEM images of electrospun nanofibers
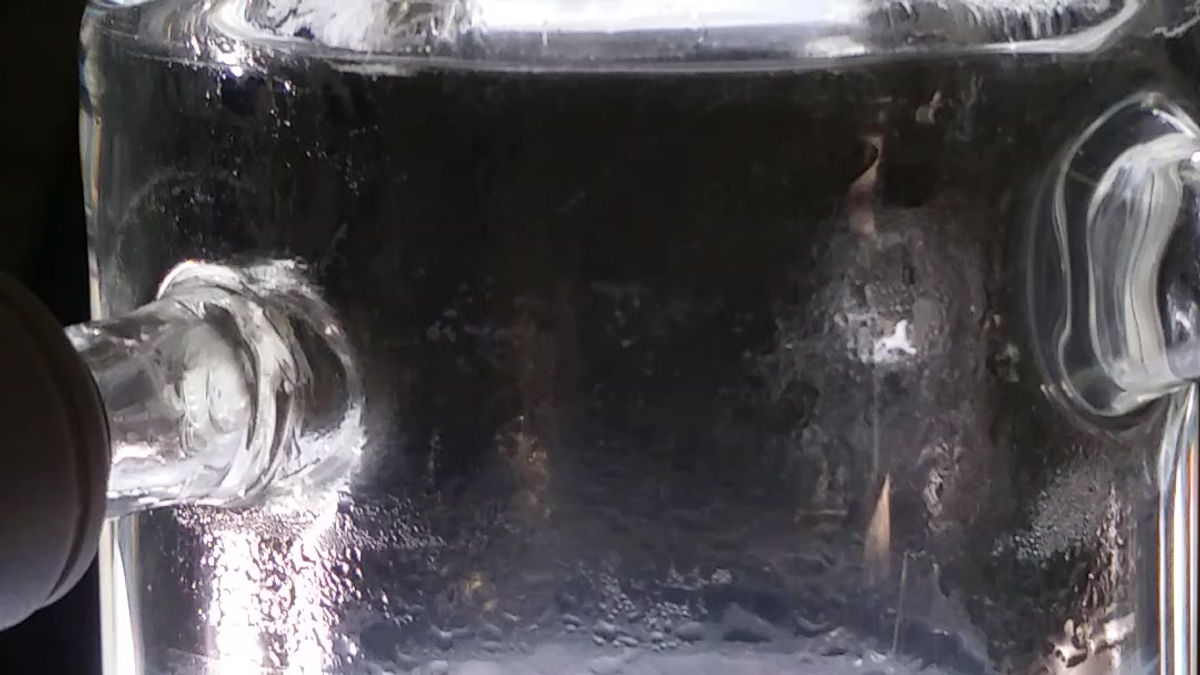
H2 generation
Video 1: H2 generation using nanofiber photocatalyst under uv-vis irradiation.
Biodiesel Synthesis:
Energy consumption and demand are ever increasing due to substantial economic growth of developing nations. Currently, fulfilling this high energy demand mainly depends on non-renewable fossil fuel resources. The unsustainability due to rapid depletion of fossil fuel reserves, environmental concerns associated with burning fossil fuels, which is the major emission source of
Chemical structure of triglycerides green-house gases such as COx, SOx, and NOx, and high demand have prompted great interest in search for renewable alternative energy sources. Renewable energy resources are environmentally benign and sustainable. Biomass conversion into usable biofuels such as biodiesel, which possesses an energy density comparable to the energy density of conventional fossil fuels, has been identified as a promising path to produce alternative fuels. Biodiesel consists of low carbon (methyl or ethyl) alkyl esters of long chain fatty acids that can be synthesized either by transesterification of methanol or ethanol with triglycerides or esterification of frees fatty acids in the presence of a catalyst. Homogeneous alkaline or acid catalysts are usually employed in transesterification reactions. However, use of homogeneous catalysis in biodiesel production has several drawbacks such as catalyst consumption during the reaction and need of vigorous purification steps, which drive up the production cost. Furthermore, most of precursor oils of biodiesel production normally contain free fatty acids (FFA) and other impurities, which pose problems during the transesterification process. FFAs tend to form soap under basic conditions and soap is known to emulsify biodiesel with glycerin, a byproduct of the transesterification reaction. It has been reported that approximately 70-90% of the total biodiesel production cost is related to the cost of raw materials and purification. Therefore, there is a pressing need to search for efficient, stable, and cost effective catalysts. Even though, heterogeneous catalysts have some advantages over homogeneous catalysts in terms of catalysts recovery and easy purification, they have their own problems such as leaching and low efficiency, which also need to be addressed. To address this problem, I will develop molecular level catalysts that are permanently attached to a support through chemical bonding. Furthermore, I will use both acid and base functionalities to the same support to carry out esterification and transesterifiction so that efficiency will be enhanced. In this part of the research, we will use acid (sulfonyl groups) and base (amino groups) functionalized organic moieties permanently supported on silica or alumina supports. Additionally, we will look into CaO (MgO) base oxides and WO3 (MoO3) superacid supported catalysts as well.
Battery Cathodes:
The total world power consumption is projected to approximately triple by year 2050. The apparent increase in world energy demand and the rapid depletion of fossil fuel resources are of concern and search of alternative sources of energy has been a focus. At present oil represents major component of world’s energy supply. Since majority of oil is being used in road transportation sector, finding alternative ways to power up vehicles is a pressing need. Currently, hybrid vehicles contribute to address above problem to some extent, but pure electric vehicles will be the best alternative. In this regard, highly efficient and high capacity batteries are required but at present, lack these kinds of batteries is a major limitation in electrifying road transportation. Interest in Li-Air batteries is growing owing to their higher gravimetric energy storage density. Apart from powering up vehicles, Li-Air batteries may have applications in other areas such as powering computers, sensors etc. The fundamental operational principle of Li-Air battery is the Li oxidation to form Li+ and electron, which travels through outer circuit to do work and finally reaches the cathode. Li+ ions travels through Li+ conducting membrane to cathode and reacts with air (O2) to form Li2O2 (and possibly Li2O). This is called discharge reaction or Oxygen Reduction Reaction (ORR). One of the drawbacks of Li-Air batteries is that the discharge product, Li2O2, gradually clogs the porous cathode affecting the performance.To enhance the performance and make Li-Air battery truly rechargeable, novel catalysts with better dispersion on support (carbon) need to be introduced. Making Li-Air batteries truly rechargeable poses significant challenges because decomposition of Li2O2 is quite challenging and efficient catalysts have to be used. Nevertheless, discharge by products need to be effectively converted into O2 and Li+ through the Oxygen Evolution Reaction (OER). Therefore, development of efficient catalysts for both ORR and OER is a timely need. In this regard, I propose to use aerosol processing technique to synthesize perovskite based bi-functional catalysts/carbon composite materials as cathode material for Li-Air batteries. Initially, perovskite materials with A1-x SrxBO3 (A = La, Ce, B=Mn, Fe, Co) composition and related compositions achieved upon doping B sites with lower oxidation state metals ions will be studied because they possess high electronic conductivity and activity towards both ORR and OER reactions. We are employing Ultrasonic Spray Pyrolysis (USP) method to synthesize porous, high surface area cathode materials (see below).
Ultrasonic Spray Pyrolysis (USP) method
Among the synthesis technologies of nanoscale materials, USP, an aerosol processing technique, has been demonstrated to be a useful tool. In this technique, a template, which has a variety of sizes, is used, and subsequent removal of template without disintegrating the structure can produce a material with high surface area and porosity, both of which can be adjusted by choosing the desired template. A precursor solution mixed with an appropriate template, SiO2 nanoparticles, carbon etc. is nebulized ultrasonically and the resultant mist (watch video) is carried by a carrier gas through a quartz tube placed in a heated furnace. The particle containing mist is allowed to condense and later collected from the water-filled collecting vessel.
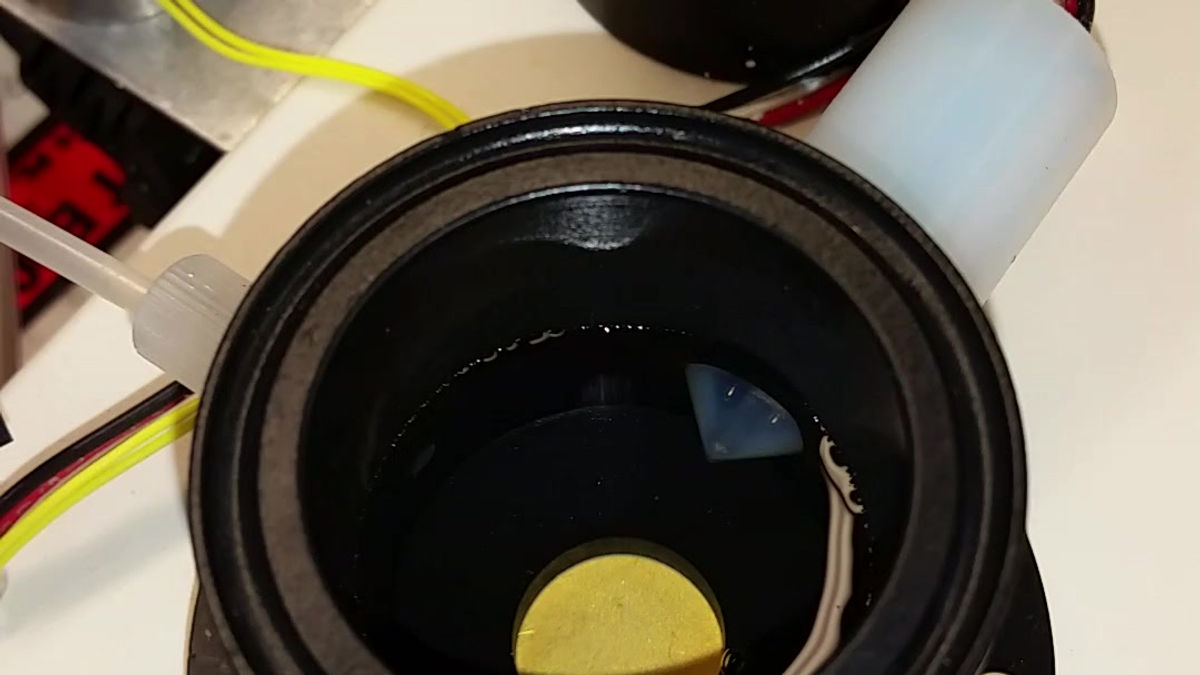
Ultrasonic nebulization
Video 2: Ultrasonic nebulization
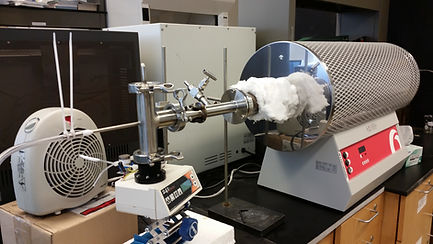
USP setup